Exploring Biomarker Technology to Enhance the Diagnosis and Treatment of PTSD in Justice-Involved Veterans
August 2024
By Soonjo Hwang, M.D., University of Nebraska Medical Center Department of Psychiatry
Key Takeaways
- PTSD rates are disproportionately high among military veterans, with as many as one third of former service members experiencing the disorder. Risk factors include multiple deployments, enlisted rank, younger age, non-White racial identity, pre-deployment trauma or mental health disorder, and low post-deployment social support.
- PTSD can elevate the risk that veterans will engage in criminal behavior, make contact with the criminal justice system, and misuse substances.
- Diagnosis of PTSD relies on self-reported symptoms, which can vary widely. Treatment options such as psychotherapy and medications are not universally effective.
- Biomarkers, which are objective and quantifiable characteristics of biological processes, offer objective measures to aid in PTSD diagnosis and treatment, potentially reducing reliance on self-reported symptoms. Biomarkers can potentially also identify high-risk individuals and be used to tailor treatment plans.
- Despite advancements, clinically actionable biomarkers for PTSD are still lacking due to the disorder’s complexity and individual variability. More research is needed, including genetic studies, long-term cohort studies, and developmental programming.
- Many biomarkers for PTSD are currently undergoing study. Machine learning can help analyze multiple biomarkers together to identify people at high risk of developing PTSD and to enable more accurate diagnosis.
- Researchers, clinicians, and policymakers should consider the ethical and legal implications of using biomarkers to diagnose PTSD or identify those at risk for developing PTSD to prevent discrimination and address privacy concerns.
This paper was produced with support from The Arthur M. Blank Family Foundation, Craig Newmark Philanthropies, The Just Trust, LinkedIn, the National Football League, T. Denny Sanford, and the Wilf Family Foundation, as well as the John D. and Catherine T. MacArthur Foundation, Southern Company Foundation, #StartSmall, and other CCJ general operating contributors.
Glossary
- Biomarkers: Objective and quantifiable characteristics of biological processes
- Brain Imaging: Application of various techniques to either directly or indirectly visualize the structure or function of the brain
- Glucocorticoid: A steroid hormone involved in body metabolism and stress response
- Hypothalamic–Pituitary–Adrenal (HPA) Axis: The fundamental system responsible for regulating stress responses and the system that regulates cortisol. Proper functioning of the HPA axis is essential for maintaining balance in the body, and dysfunction in this system can contribute to various health issues, including PTSD, anxiety, and depression.
- Machine Learning: A branch of artificial intelligence that focuses on development of algorithms driven directly from data without explicit instructions
- Neurotransmitters: Chemical messengers that brain cells use to communicate with each other
- Post-Traumatic Stress Disorder (PTSD): A psychiatric disorder that can develop after exposure to a traumatic event
- Psychotherapy: A type of treatment in which behavioral, cognitive, and emotional techniques are applied
- Psychotropic Medication: Any medication that can affect the brain’s cognitive, emotional, and decision-making functions
- Sensitivity and Specificity: Terms used to describe the ability of a test to correctly identify a person with a disorder as positive (sensitivity) or to correctly identify a person without a disorder as negative (specificity)
Introduction
Post-traumatic stress disorder (PTSD) is a psychiatric disorder that may occur after a traumatic life experience.1 Common PTSD symptoms include re-experiencing the traumatic experience, the avoidance of trauma-related stimuli, hyperarousal, and negative alteration (or change) of thoughts and emotion. Related symptoms include aggression, impulsivity, hypervigilance, misappraisal of threat, sensation seeking, fear, anxiety, depression, and suicidal thoughts and behaviors.2 The overall lifetime prevalence of PTSD among the general public is 6.1%, and PTSD rates are higher among women (8%) than men (4.1%).3 PSTD is difficult to accurately diagnose and treat; diagnosis relies on self-reported symptoms, and existing psychotherapy and pharmacological treatments are not always effective for people with PTSD.
Prevalence of PTSD Among Military Veterans
PTSD prevalence rates are elevated among military service members and veterans. Eight percent of male veterans and 13% of female veterans have PTSD, compared to 3% of male civilians and 8% of female civilians.4 Among veterans who experienced combat and other traumatic events during their service, lifetime PTSD prevalence may be considerably higher, up to 29%.5 Veterans with multiple deployments are three times more likely than those who were not deployed to be diagnosed with PTSD.6 Other risk factors for the development of PTSD among veterans include lower (or junior) enlisted rank, younger age, non-White racial identity, female gender, a history of pre-deployment trauma or mental health disorder, and low/absent post-deployment social support—factors disproportionately prevalent among post-9/11 veterans.7 As of September 2023, 1.5 million of the estimated total 18.1 million veterans were receiving compensation for service-connected PTSD.8 Of the six million veterans who accessed healthcare from the U.S. Department of Veterans Affairs (VA) in 2021, 19% of women and 10% of men were diagnosed with PTSD.9
PTSD with Criminal Justice System Involvement
Although not every veteran who experiences trauma or PTSD engages in criminal behavior, deployment-related trauma exposure, combined with increased incidence of mental health and substance use disorders, elevate the risk for veterans to misuse substances, engage in criminal behavior, and make contact with the justice system.10
Researchers, however, have rarely explored how trauma exposure and PTSD connect to criminal behavior for veterans.11 Veterans, and especially veterans with PTSD, are more likely to self-harm or engage in non-suicidal self-injury than non-veterans. Estimates suggest that 4% to 6% of non-veterans engage in self-harm, compared to 3% to 14% of veterans and 57% of veterans seeking treatment for PTSD.12 Incarcerated male veterans are also significantly more likely than incarcerated male non-veterans to have committed a violent offense. For example, in 2016—the most recent available data—69% of veterans in state prison and 25% in federal prison were convicted of a violent offense compared to 57% of non-veterans in state prison and 13% in federal prison.13
The risk for veterans with PTSD to engage in violent behaviors and suicide is increased when combined with other factors, such as severity of anger or alcohol misuse.14 In addition, analyses show that the odds of criminal justice system involvement are 61% greater for veterans with PTSD than for those without PTSD; the odds of arrest for violent offenses are 59% greater for those with PTSD.15
Challenges to PTSD Diagnosis and Treatment
Clinicians face several challenges in accurately diagnosing and treating PTSD, which in turn reduces the likelihood of effective intervention. Diagnosis currently relies on self-reported symptoms and the clinician’s judgment, and reliability rates are low, meaning that clinicians do not always agree about who is experiencing PTSD and who is not. Clinical diagnosis requires an individual to report at least six of the 20 PTSD symptoms outlined in the Diagnostic and Statistical Manual of Mental Disorders (Fifth Ed.). Because of this requirement, two people diagnosed with PTSD may not have any overlap in their symptoms.16 This extreme individual variation has complicated the search for prevention mechanisms and effective PTSD treatments.17
Additionally, people who are experiencing PTSD do not always understand what is happening to them or see that changes in their thoughts and behaviors are part of the experience of PTSD. Service members and veterans may underreport symptoms due to the stigma around mental health issues in the military or may overreport as an attempt to be discharged or receive financial compensation.18
Treatment for PTSD relies on psychotherapy and medications, neither of which are universally effective for all patients with the disorder.19 The evidence-based treatment with the highest effectiveness is trauma-focused psychotherapy, a standard treatment for PTSD patients whose care is provided by the VA. Although more than half of military-related PTSD patients showed clinically meaningful symptom improvement after receiving this psychotherapy treatment (defined as a 10- to 12-point decrease in PTSD symptoms using a validated measure), these decreases weren’t enough to move patients’ PTSD scores out of the clinical range.20 Instead, between half and three-quarters of patients who saw symptom improvement through trauma-focused psychotherapy treatment retained the PTSD diagnosis.
How the Identification of Biomarkers Can Improve PTSD Diagnosis and Treatment
Biomarkers are a wide umbrella of objective and biological measures that inform patients and clinicians about the patient’s biological processes.21 Identifying reliable biomarkers may mitigate common PTSD diagnostic and treatment issues, moving diagnosis and treatment away from self-reported symptoms.22 This would allow clinicians to have an objective measure of PTSD to use for diagnosis and prediction of clinical outcomes. In this way, a clinician could test a patient for PTSD using saliva, blood, or some other biological marker in the same way doctors test patients for the flu.
Once clear biomarkers are identified, they have the potential to be used by clinicians to identify those at high risk for PTSD and create and test prevention strategies to reduce the number of veterans who develop PTSD. During treatment, biomarkers could potentially be used to match patients to specific medications, increasing effectiveness and reducing adverse effects. During pharmacotherapy, biomarkers could potentially be used to measure treatment effects (positive or negative) and refine the clinical plan. Although clinically actionable biomarkers for PTSD have not yet been identified, this review highlights the biomarkers that have shown the most potential for improving PTSD diagnosis and treatment.
Peripheral Biomarkers: Exploring the “Fight or Flight” Response
Peripheral biomarkers are obtained from the periphery of—or away from—the brain, including blood, saliva, and urine. For example, the stress hormone cortisol can be obtained from blood, saliva, urine, and cerebrospinal fluid. While many peripheral biomarker candidates are being researched, the stress hormone cortisol and the Hypothalamic-Pituitary-Adrenal (HPA) axis, a fundamental system responsible for regulating stress responses and the system that regulates cortisol, have received the most research attention. A neurotransmitter called norepinephrine, or noradrenaline, that regulates arousal, attention, and stress responses, has also received substantial research attention.
Cortisol and HPA Axis
The HPA axis is a three-organ system that releases cortisol, the body’s primary stress hormone. When we perceive a threat, the hypothalamus (H), pituitary gland (P), and adrenal glands (A) work together to release a surge of hormones, including cortisol (see Figure 1).23 Cortisol releases sugar from the liver for fast energy during times of stress and lets the body focus on survival by slowing nonessential functions.24 When functioning normally, cortisol hormones return to their baseline levels, and all other systems resume functioning once the threat has passed.
Figure 1. Regulation of Cortisol Secretion in the HPA Axis
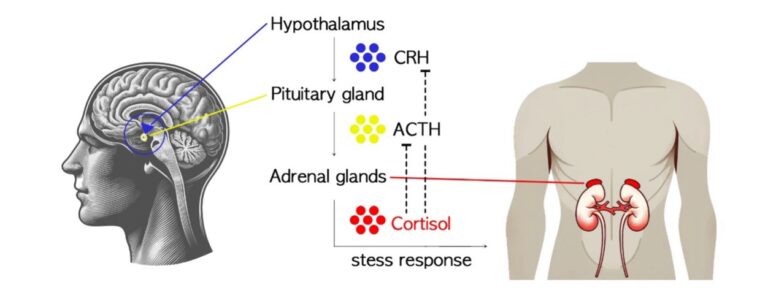
Though there are several other important stress hormones, cortisol is strongly correlated to PTSD, with many studies showing abnormal changes in cortisol related to the increased sensitivity of the HPA axis.25 Many symptoms of PTSD can be understood as an expression of a chronic “fight or flight” response. In general, fight or flight responses are accompanied by high cortisol levels and result in enhanced consolidation of fear memory. Paradoxically, low cortisol levels are also observed in some patients with PTSD; these are understood as an increased negative feedback inhibition via increased glucocorticoid receptor sensitivity at the pituitary level in the HPA axis. That is, when someone is experiencing PTSD, their brain and body perceive danger—and react as though they are in danger—long after the dangerous situation has ended.
The Neurotransmitter Norepinephrine
Like cortisol, norepinephrine is part of the fight or flight response of the autonomic nervous system. Norepinephrine is a neurotransmitter, a chemical produced in the body that allows nerve cells to communicate with each other. Also called noradrenaline, norepinephrine is one of the most researched neurotransmitters for PTSD. It is produced in the brainstem and near the spinal cord, regulating arousal, attention, cognitive function, and stress reactions.
Research conducted with samples of people experiencing PTSD consistently find elevated levels of norepinephrine in the blood or cerebrospinal fluid. These high levels of norepinephrine impair attention, decrease working memory, and are associated with the intrusive re-experiencing of traumatic memories.26
Genetic Biomarkers: Using Genes for Prevention, Diagnosis, and Treatment
Many genes have been studied and have shown significant findings in connection with PTSD. Among those gene findings, NR3C1 and FKBP5 have shown significant associations with PTSD symptom severity as well as recovery and resilience.27 Ultimately, the genes that regulate cortisol level and the HPA axis may serve as biomarkers for the risk of developing PTSD, diagnosis of PTSD, and the course of the disease.
NR3C1
The NR3C1 gene is responsible for the receptor of cortisol and other stress hormones, which plays a pivotal role in how well the HPA axis functions to release hormones overall.28 Heightened receptors of cortisol and other glucocorticoids increase susceptibility to PTSD.
Research shows that people with PTSD have higher levels of NR3C1 gene activity compared to those without the condition.29 This phenomenon is pronounced in individuals who have recently undergone traumatic experiences and are grappling with the acute manifestations of PTSD.
FKBP5
The FKBP5 gene affects how sensitive the body’s glucocorticoid receptors are to cortisol and other stress hormones.30 This gene can predict changes in brain structure and dysregulated stress hormone receptor signaling in people with PTSD.
Studies have shown that people with PTSD who have experienced child abuse display higher sensitivity to stress hormones due to the specific single nucleotide changes in the FKBP5 gene, unlike those who have experienced child abuse but do not have PTSD.31 When trauma occurs, it can activate a specific form of FKBP5, leading to resistance to stress hormones and prolonged stress responses, which could increase the risk for the development of PTSD symptoms.
Genetic biomarker discoveries can help improve PTSD treatment by identifying new targets for psychotropic medication and potentially identifying subgroups of people with a specific genetic profile who might be the most likely to benefit from a pharmaceutical or other treatment. Genetic biomarkers may also allow for the identification of those at high risk for developing PTSD, helping them to avoid trauma exposure and subsequent PTSD.32
Neuroimaging Biomarkers: How Brain Scans Can Facilitate PTSD Diagnosis
Neuroimaging refers to a range of non-invasive techniques that study brain structure and function. These techniques can be categorized into two approaches: structural and functional. Structural neuroimaging involves specialized methods for visualizing and analyzing the anatomical properties of the brain. Common techniques in this category include magnetic resonance imaging (MRI) and computed tomography (CT) scans, which provide detailed images of brain structures.33 Functional neuroimaging is used to identify brain regions and underlying processes associated with specific tasks or functions. Techniques such as functional MRI, positron emission tomography (PET) scan, magnetic resonance spectroscopy, and electroencephalography (EEG) capture brain activity and connectivity patterns during various cognitive and behavioral tasks.34
It is hoped that these neuroimaging approaches can identify the areas of the brain and specific functions that are involved in the disease process of PTSD. For example, several studies have reported reduced volumes of certain parts of the brain in people diagnosed with PTSD compared to people who do not have PTSD35 Research also shows that as the volumes of these brain regions decrease, the severity of PTSD symptoms tends to increase.36 Additionally, a subgroup of people with PTSD showed stronger neural connections between parts of the brain implicated in emotion regulation, consciousness, awareness, and proprioception (also known as kinesthesia, which is a sense that helps us know where our body parts are and how they are moving without needing to look at them) compared to those with PTSD who were not also experiencing disassociation .37 Finally, when compared to people who experienced trauma but who do not have PTSD and to people with PTSD alone, those with PTSD and major depressive disorder showed fewer connections in a key brain network involved in fear processing and in two pathways related to reward processing.38
Physiological Biomarkers: Using Body Reflexes to Diagnose PTSD
Physiological reflexes vary between people with PTSD and people who have experienced trauma but do not have PTSD. These differences suggest that physiological biomarkers could help clinicians more quickly and effectively diagnose PTSD to ensure people receive treatment.
Studies show, for example, that an increased heart rate after exposure to traumatic events is also associated with developing PTSD.39 People already diagnosed with PTSD have heightened heart rate responses to emotional stimuli, as well as reduced reactions to aversive pictures (sometimes described as the “freezing” response to threat).40 People with PTSD also exhibit other differences, including heightened startle reflexes.41 Heart rate variability, the change in heart rate that occurs when someone is exposed to threat, is an example of an autonomic response. People with PTSD often show decreased heart rate variability, meaning that this autonomic response is not functioning as expected.42 It is unclear whether reduced heart rate variability is associated with risk for the development of PTSD, or whether it is a consequence of PTSD.
Machine Learning Approaches: Searching for Multiple Biomarkers
It is unlikely that a single biomarker for PTSD will be identified, given PTSD’s complexity, individual variability, the intricate biological mechanisms underlying the disorder, and the likelihood that people with PTSD have other mental health or substance use disorders.43 The analysis of numerous biomarkers, therefore, may benefit from machine learning approaches. Machine learning is a subset of artificial intelligence designed to empower computers to autonomously learn and adapt, increasing precision and effectiveness through iteration.44 Machine learning approaches may be able to predict the likelihood of PTSD onset following a traumatic incident, diagnose PTSD based on initial post-traumatic responses, or predict prognosis.45
To enhance the specificity and sensitivity of diagnostic tools, biomarker panels composed of diverse data types are imperative. These panels encompass DNA, RNA, protein, metabolite, brain imaging, and clinical data, with machine learning serving as a pivotal tool for integrating and analyzing these multi-layered datasets. For example, a diagnostic biomarker panel comprising 28 biomarkers derived from male veterans with combat-related PTSD and veterans who experienced combat but who did not develop PTSD demonstrated promising metrics for PSTD identification.46 This approach, while still in its infancy, highlights a compelling trajectory for research advancing the development of PTSD biomarkers, leveraging the power of biomarker panels and machine learning methodologies.
Machine learning can provide insight into the complex relations of various biomarkers without an already determined theory or hypothesis. Artificial intelligence can provide a tool to achieve this goal.
Clinicians Need More Research Before Using Biomarkers to Diagnose and Treat PTSD
Despite significant efforts and investments made over the past few decades, researchers have been unable to identify clinically actionable biomarkers for PTSD.47 The broad variation in the symptoms experienced by people with PTSD poses a substantial challenge to biomarker identification.48 Other factors complicating the identification of one or more definitive PTSD biomarkers include the tendency for people with PTSD to have additional mental health and substance use disorders, as well as general medical conditions such as hypertension, cardiovascular disease, and inflammatory conditions.49
Also, most psychiatric research has relied on case-control designs, which are unlikely to result in the identification of actionable biomarkers.50 The intricate interplay of biological diversity, high comorbidity, complex pathophysiology, and study design challenges further complicates the quest for diagnostic biomarkers for PTSD.
Several key actions can help improve our ability to find biomarkers for PTSD. First, genetic studies involving large groups of patients should be conducted. These studies aid in understanding how both genes and environmental factors contribute to PTSD development and treatment. Additionally, a focus on specific symptoms or groups of symptoms experienced by people with PTSD may provide potential variations in treatment approaches.
Long-term studies tracking PTSD patients over time can also play a crucial role in the identification of biomarkers. These types of studies provide valuable information about how the disorder evolves and the effectiveness of treatments over time. Additionally, researchers should use stratification methods to categorize individuals based on their biological risk factors. Stratification methods are techniques used to divide participants into groups based on specific characteristics, such as the type of trauma-exposure, genetics, age, sex, or other health indicators. This approach will facilitate testing treatments tailored to a patient’s unique needs.
Researchers should also take pains to consider factors like sex and gender or adverse childhood experiences when designing studies.51 Greater understanding of how these factors contribute to the development of PTSD and how they may be leveraged to identify targeted treatment strategies is needed.
The Ethical and Legal Implications of Biomarkers
If and when biomarkers are identified, they might be used to determine whether a person is at high risk for developing PTSD and/or how a patient might be likely to benefit from one or more tailored treatment approaches. For military service members, this might mean undergoing screening for PTSD susceptibility pre-deployment, or that biomarkers are used to evaluate service members as they return home.52 Biomarkers may be used to develop tailored treatments for military service members and veterans.53 While these approaches hold promise for helping to connect people with PTSD to needed services, biomarkers do not account for resilience or the fact that not all people who are exposed to trauma develop PTSD.
Additionally, identified biomarkers may have low specificity, meaning that some people may be identified with PTSD when they do not have it (false positives). Conversely, biomarkers may have low sensitivity, meaning some people may not be identified as having PTSD when they do (false negatives). These incorrect designations may lead screened service members to receive (or not receive) clinical diagnosis and treatment. Further, even a highly accurate biomarker test may help craft effective clinical treatment, but will likely remain inadequate for legal purposes should use it as evidence of a service-connected disability for PTSD.54
As the pursuit of PTSD biomarkers continues, a parallel conversation regarding ethical and legal implications is needed. Currently, biomarkers do not predict the most serious outcomes of PTSD—violent behavior and death by suicide—risks that are elevated for veterans. Future research should focus on these areas to speed biomarker development that can be used to prevent violence and save lives. Clinicians, especially those who work directly with military service members and veterans, should stay abreast of neurobiological and neuroscientific research to include innovative findings into their assessment and treatment plans. At a minimum, the role that biomarkers play in the development of PTSD can help shift the enduring and damaging perception of PTSD as a “character flaw” or lack of resiliency among service members and veterans who develop the disorder.
Review of PTSD Biomarker Research Findings
TYPES OF BIOMARKER
LITERATURE FINDINGS
PERIPHERAL BIOMARKERS
Cortisol reactivity to an experimental stressor
Blunted cortisol response predicted chronic increasing distress patterns.55
Bedtime salivary cortisol level and 24-hour urinary cortisol excretion
Higher bedtime salivary cortisol and 24-hour urinary cortisol excretion predicted treatment response to prolonged exposure therapy.56
Cortisol awakening response
Slower cortisol awakening response following dexamethasone administration predicted greater PTSD symptom decrease in trauma-focused psychotherapy.57
Cortisol levels after reading trauma script
The increased cortisol response to reading a personal trauma script prior to PTSD therapy was significantly related to reductions in the core PTSD symptoms in prolonged exposure therapy.58
GENETIC BIOMARKERS
Overexpression of NR3C1 gene (glucocorticoid receptor gene)
The AUC of NR3C1 expression was 0.797, and the sensitivity and specificity were 62.5% and 89.8%, respectively.59
FKBP5 (regulate glucocorticoid receptor sensitivity) genetic variants
Individuals who carry FKBP5 genetic variants exposed to early-life trauma had a higher risk for PTSD.60
Polymorphism of SLC6A3 (dopamine transporter gene)
Polymorphism of SLC6A3 (dopamine transporter gene) showed a significant association with PTSD (OR=1.62).61
Polymorphism of DRD2 (dopamine D2 receptor gene)
rs1800497 in DRD2 was significantly associated with PTSD (OR=1.96).62
Differential methylation within NGR1 and HGS
rs2267335 within ADCYAP1R1 (PACAP gene) predicted PTSD diagnosis in females only.63
rs2267335 within ADCYAP1R1 (PACAP gene) (only in females)
Differential methylation within NGR1 and HGS was significantly associated with current PTSD across three civilian cohorts.64
NEUROIMAGING BIOMARKERS
Amygdala volume
Reductions in amygdala volume were related to post-traumatic stress.65
Left hippocampal volume
A negative correlation was found between total PTSD score and left hippocampal volume and between re-experiencing subscale of PTSD and left hippocampal volume.66
Lateral orbitofrontal gyrus volume
Reduced volumes of left and right lateral orbitofrontal gyri were negatively correlated with post-traumatic stress symptom severity.67
Amygdala functional connectivity to prefrontal regions
People experiencing the dissociative subtype of PTSD showed greater amygdala functional connectivity to prefrontal regions compared with the PTSD without dissociative subtype group.68
Connectivity in basolateral amygdala-orbitofrontal cortex, nucleus accumbens-thalamus, and nucleus accumbens-hippocampus
People with PTSD and major depressive disorder exhibited greater decreases in connectivity in both the basolateral amygdala-orbitofrontal cortex (a fear-processing network) and in two striatal-subcortical pathways that play a role in reward processing (the nucleus accumbens-thalamus and nucleus accumbens-hippocampus).69
PHYSIOLOGICAL BIOMARKERS
Endnotes
1 American Psychiatric Association. (2013). Diagnostic and statistical manual of mental disorders (5th ed.). https://doi.org/10.1176/appi.books.9780890425596
2 Ibid.
3 Goldstein, R. B., Smith, S. M., Chou, S. P., Saha, T. D., Jung, J., Zhang, H., … & Grant, B. F. (2016). The epidemiology of DSM-5 posttraumatic stress disorder in the United States: Results from the National Epidemiologic Survey on Alcohol and Related Conditions-III. Social Psychiatry & Psychiatric Epidemiology, 51(8), 1137-1148. https://link.springer.com/article/10.1007/s00127-016-1208-5
4 Lehavot, K., Katon, J. G., Chen, J. A., Fortney, J. C., & Simpson, T. L. (2018). Post-traumatic stress disorder by gender and veteran status. American Journal of Preventive Medicine, 54(1), e1-e9. https://doi.org/10.1016/j.amepre.2017.09.008
5 Na, P. J., Schnurr, P. P., & Pietrzak, R. H. (2023). Mental health of U.S. combat veterans by war era: Results from the national health and resilience in veterans study. Journal of Psychiatric Research, 158, 36-40. https://doi.org/10.1016/j.jpsychires.2022.12.019
6 Kline, A., Falca-Dodson, M., Sussner, B., Ciccone, D. S., Chandler, H., Callahan, L., & Losonczy, M. (2010). Effects of repeated deployment to Iraq and Afghanistan on the health of New Jersey Army National Guard troops: Implications for military readiness. American Journal of Public Health, 100(2), 276-283. https://doi.org/10.2105/AJPH.2009.162925
7 Wall, P. L. (2012). Posttraumatic stress disorder and traumatic brain injury in current military populations: A critical analysis. Journal of the American Psychiatric Nurses Association, 18(5), 278-298. https://doi.org/10.1177/1078390312460578; Peterson, A. L., Luethcke, C. A., Borah, E. V., Borah, A. M., & Young-McCaughan, S. (2011). Assessment and treatment of combat-related PTSD in returning war veterans. Journal of Clinical Psychology in Medical Settings, 18(2), 164-175. https://doi.org/10.1007/s10880-011-9238-3
8 National Center for Veterans Analysis and Statistics. (2023). VA benefits & health care utilization. U.S. Department of Veterans Affairs. https://www.va.gov/VETDATA/docs/pocketcards/fy2023q4_alteration.pdf ; U.S. Department of Veterans Affairs. (2023). Veterans day 2023. https://www.data.va.gov/stories/s/Veterans-Day-2023/4gqv-ddx7/#:~:text=This%20Veterans%20Day%2C%20we%20explore,in%20FY%202023%20(2)
9 Greenberg, C., Hoff, R. (2022). FY 2021 veterans with PTSD data sheet: National, VISN, and healthcare system tables. Northeast Program Evaluation Center. https://www.ptsd.va.gov/professional/treat/essentials/epidemiology.asp
10 Cusack, M., & Montgomery, A. E. (2017). Examining the bidirectional association between veteran homelessness and incarceration within the context of permanent supportive housing. Psychological Services, 14(2), 250-256. http://dx.doi.org/10.1037/ser0000110; Finlay, A. K., Owens, M. D., Taylor, E., Nash, A., Capdarest-Arest, N., Rosenthal, J., … & Timko, C. (2019). A scoping review of military veterans involved in the criminal justice system and their health and healthcare. Health & Justice, 7(1), 1-18. https://doi.org/10.1186/s40352-019-0086-9; Logan, M. W., McNeeley, S., & Morgan, M. A. (2021). The effects of traumatic brain injury and post-traumatic stress disorder on prison adjustment and recidivism among military veterans: evidence from Minnesota. Psychiatric Quarterly, 92(3), 1147-1158. https://link.springer.com/article/10.1007/s11126-021-09883-1; Tsai, J., Rosenheck, R. A., Kasprow, W. J., & McGuire, J. F. (2014). Homelessness in a national sample of incarcerated veterans in state and federal prisons. Administration & Policy in Mental Health & Mental Health Services Research, 41(3), 360-367. https://link.springer.com/article/10.1007/s10488-013-0483-7
11 Ardino, V. (2012). Offending behaviour: The role of trauma and PTSD. European Journal of Psychotraumatology, 3(1), 18968. https://doi.org/10.3402/ejpt.v3i0.18968
12 Kimbrel, N. A., DeBeer, B. B., Meyer, E. C., Gulliver, S. B., & Morissette, S. B. (2016). Nonsuicidal self-injury and suicide attempts in Iraq/Afghanistan war veterans. Psychiatry Research, 243, 232-237. https://doi.org/10.1016/j.psychres.2016.06.039
13 Maruschak, L. M., Bronson, J., & Alper, M. (2021). Survey of prison inmates, 2016: Veterans in prison
(NCJ 252646). Bureau of Justice Statistics. https://bjs.ojp.gov/content/pub/pdf/vpspi16st.pdf
14 Barr, N., Kintzle, S., Sullivan, K., & Castro, C. (2018). Suicidality and nonsuicidal high-risk behavior in military veterans: How does PTSD symptom presentation relate to behavioral risk? Traumatology, 24(1), 55. https://doi.org/10.1037/trm0000133; Miles, S. R., Menefee, D. S., Wanner, J., Teten Tharp, A., & Kent, T. A. (2016). The relationship between emotion dysregulation and impulsive aggression in veterans with posttraumatic stress disorder symptoms. Journal of Interpersonal Violence, 31(10), 1795-1816. https://doi.org/10.1177/0886260515570746
15 Taylor, E. N., Timko, C., Nash, A., Owens, M. D., Harris, A. H. S., & Finlay, A. K. (2020). Posttraumatic stress disorder and justice involvement among military veterans: A systematic review and meta-analysis. Journal of Traumatic Stress, 33(5), 804–812. https://doi.org/10.1002/jts.22526
16 Galatzer-Levy, I. R., & Bryant, R. A. (2013). 636,120 ways to have posttraumatic stress disorder. Perspectives on Psychological Science, 8(6), 651-662. https://doi.org/10.1177/1745691613504115
17 Hoge, C. W., Yehuda, R., Castro, C. A., McFarlane, A. C., Vermetten, E., Jetly, R., … & Rothbaum, B. O. (2016). Unintended consequences of changing the definition of posttraumatic stress disorder in DSM-5: Critique and call for action. JAMA Psychiatry, 73(7), 750-752. https://jamanetwork.com/journals/jamapsychiatry/article-abstract/2524846
18 Frueh, B. C., Gold, P. B., & de Arellano, M. A. (1997). Symptom overreporting in combat veterans evaluated for PTSD: Differentiation on the basis of compensation seeking status. Journal of Personality Assessment, 68(2), 369-384. https://doi.org/10.1207/s15327752jpa6802_8; Sharp, M.-L., Fear, N. T., Rona, R. J., Wessely, S., Greenberg, N., Jones, N., & Goodwin, L. (2015). Stigma as a barrier to seeking health care among military personnel with mental health problems. Epidemiologic Reviews, 37(1), 144-162. https://doi.org/10.1093/epirev/mxu012
19 Bisson, J. I., Berliner, L., Cloitre, M., Forbes, D., Jensen, T. K., Lewis, C., Monson, C. M., Olff, M., Pilling, S., & Riggs, D. S. (2019). The international society for traumatic stress studies new guidelines for the prevention and treatment of posttraumatic stress disorder: Methodology and development process. Journal of Traumatic Stress, 32(4), 475-483. https://doi.org/10.1002/jts.22421; Bradley, R., Greene, J., Russ, E., Dutra, L., & Westen, D. (2005). A multidimensional meta-analysis of psychotherapy for PTSD. American Journal of Psychiatry, 162(2), 214-227. https://doi.org/10.1176/appi.ajp.162.2.214; Lee, D. J., Schnitzlein, C. W., Wolf, J. P., Vythilingam, M., Rasmusson, A. M., & Hoge, C. W. (2016). Psychotherapy versus pharmacotherapy for posttraumatic stress disorder: Systematic review and meta‐analyses to determine first‐line treatments. Depression & Anxiety, 33(9), 792-806. https://doi.org/10.1002/da.22511
20 Steenkamp, M. M., Litz, B. T., Hoge, C. W., & Marmar, C. R. (2015). Psychotherapy for military-related PTSD: A review of randomized clinical trials. JAMA, 314(5), 489-500. https://doi.org/10.1001/jama.2015.8370; Straud, C. L., Siev, J., Messer, S., & Zalta, A. K. (2019). Examining military population and trauma type as moderators of treatment outcome for first-line psychotherapies for PTSD: A meta-analysis. Journal of Anxiety Disorders, 67, 102133. https://doi.org/10.1016/j.janxdis.2019.102133
21 FDA-NIH Biomarker Working Group. (2016). In BEST (Biomarkers, EndpointS, and other Tools) Resource. https://www.ncbi.nlm.nih.gov/pubmed/27010052
22 López-Ojeda, W., & Hurley, R. A. (2022). Extended reality technologies: Expanding therapeutic approaches for PTSD. The Journal of Neuropsychiatry & Clinical Neurosciences, 34(1), A4-5. https://doi.org/10.1176/appi.neuropsych.21100244; Wu, Y., Mao, K., Dennett, L., Zhang, Y., & Chen, J. (2023). Systematic review of machine learning in PTSD studies for automated diagnosis evaluation. NPJ Mental Health Research, 2(1), 16. https://doi.org/10.1038/s44184-023-00035-w
23 Chu, B., Marwaha, K., Sanvictores, T., & Ayers, D. (2022). Physiology, stress reaction. StatPearls Publishing. https://www.ncbi.nlm.nih.gov/books/NBK541120/
24 Thau, L., Gandhi, J., & Sharma, S. (2023). Physiology, cortisol. StatPearls Publishing. https://www.ncbi.nlm.nih.gov/books/NBK538239/
25 Bryant, R. A. (2019). Post‐traumatic stress disorder: A state‐of‐the‐art review of evidence and challenges. World Psychiatry, 18(3), 259-269. https://doi.org/10.1002/wps.20656; Pan, X., Kaminga, A. C., Wen, S. W., Wang, Z., Wu, X., & Liu, A. (2020). The 24-hour urinary cortisol in post-traumatic stress disorder: A meta-analysis. PLoS One, 15(1), e0227560. https://doi.org/10.1371/journal.pone.0227560; Yehuda, R., Southwick, S. M., Nussbaum, G., Wahby, V., Giller, E. L., & Mason, J. W. (1990). Low urinary cortisol excretion in patients with posttraumatic stress disorder. The Journal of Nervous & Mental Disease, 178(6), 366-369. https://doi.org/10.1097/00005053-199006000-00004
26 Bryant, R. A. (2019). Post‐traumatic stress disorder: A state‐of‐the‐art review of evidence and challenges. World Psychiatry, 18(3), 259-269. https://doi.org/10.1002/wps.20656; Hendrickson, R. C., & Raskind, M. A. (2016). Noradrenergic dysregulation in the pathophysiology of PTSD. Experimental Neurology, 284, 181-195. https://doi.org/10.1016/j.expneurol.2016.05.014; Southwick, S. M., Krystal, J. H., Bremner, J. D., Morgan, C., 3rd, Nicolaou, A. L., Nagy, L. M., Johnson, D. R., Heninger, G. R., & Charney, D. S. (1997). Noradrenergic and serotonergic function in posttraumatic stress disorder. Archives of General Psychiatry, 54(8), 749-758. https://doi.org/10.1001/archpsyc.1997.01830200083012; Southwick, S. M., Krystal, J. H., Morgan, C. A., Johnson, D., Nagy, L. M., Nicolaou, A., Heninger, G. R., & Charney, D. S. (1993). Abnormal noradrenergic function in posttraumatic stress disorder. Archives of General Psychiatry, 50(4), 266-274. https://doi.org/10.1001/archpsyc.1993.01820160036003
27 Sheerin, C. M., Lind, M. J., Bountress, K. E., Marraccini, M. E., Amstadter, A. B., Bacanu, S. A., & Nugent, N. R. (2020). Meta‐analysis of associations between hypothalamic‐pituitary‐adrenal axis genes and risk of posttraumatic stress disorder. Journal of Traumatic Stress, 33(5), 688-698. https://doi.org/10.1002/jts.22484
28 Watkeys, O. J., Kremerskothen, K., Quidé, Y., Fullerton, J. M., & Green, M. J. (2018). Glucocorticoid receptor gene (NR3C1) DNA methylation in association with trauma, psychopathology, transcript expression, or genotypic variation: A systematic review. Neuroscience & Biobehavioral Reviews, 95, 85-122. https://doi.org/10.1016/j.neubiorev.2018.08.017
29 Ramírez, C. G., Queijeiro, A. V., Morales, S. J., López, D. B., Miranda, A. H., Chow, A. R., Cárdenas, L. T., & Estrada, M. G. (2020). The NR3C1 gene expression is a potential surrogate biomarker for risk and diagnosis of posttraumatic stress disorder. Psychiatry Research, 284, 112797. https://doi.org/10.1016/j.psychres.2020.112797
30 Zannas, A. S., Wiechmann, T., Gassen, N. C., & Binder, E. B. (2016). Gene–stress–epigenetic regulation of FKBP5: Clinical and translational implications. Neuropsychopharmacology, 41(1), 261-274. https://doi.org/10.1038/npp.2015.235
31 Binder, E. B. (2009). The role of FKBP5, a co-chaperone of the glucocorticoid receptor in the pathogenesis and therapy of affective and anxiety disorders. Psychoneuroendocrinology, 34, S186-S195. https://doi.org/10.1016/j.psyneuen.2009.05.021
32 Smoller, J. W. (2016). The genetics of stress-related disorders: PTSD, depression, and anxiety disorders. Neuropsychopharmacology, 41(1), 297-319. https://doi.org/10.1038/npp.2015.266
33 Brammer, M. (2009). The role of neuroimaging in diagnosis and personalized medicine-current position and likely future directions. Dialogues in Clinical Neuroscience, 11(4), 389-396. https://doi.org/10.31887/DCNS.2009.11.4/mbrammer
34 Brammer, M. (2009). The role of neuroimaging in diagnosis and personalized medicine-current position and likely future directions. Dialogues in Clinical Neuroscience, 11(4), 389-396. https://doi.org/10.31887/DCNS.2009.11.4/mbrammer
35 These are the hippocampus, amygdala, dorsomedial prefrontal cortex, and ventromedial prefrontal cortex. See: Ganzel, B. L., Kim, P., Glover, G. H., & Temple, E. (2008). Resilience after 9/11: Multimodal neuroimaging evidence for stress-related change in the healthy adult brain. Neuroimage, 40(2), 788-795. https://doi.org/10.1016/j.neuroimage.2007.12.010; Li, L., Wu, M., Liao, Y., Ouyang, L., Du, M., Lei, D., Chen, L., Yao, L., Huang, X., & Gong, Q. (2014). Grey matter reduction associated with posttraumatic stress disorder and traumatic stress. Neuroscience & Biobehavioral Reviews, 2014(43), 163-172. https://doi.org/10.1016/j.neubiorev.2014.04.003; Logue, M. W., van Rooij, S. J., Dennis, E. L., Davis, S. L., Hayes, J. P., Stevens, J. S., Densmore, M., Haswell, C. C., Ipser, J., & Koch, S. B. (2018). Smaller hippocampal volume in posttraumatic stress disorder: A multisite ENIGMA-PGC study: Subcortical volumetry results from posttraumatic stress disorder consortia. Biological Psychiatry, 83(3), 244-253. https://doi.org/10.1016/j.biopsych.2017.09.006; Rauch, S. L., Shin, L. M., Segal, E., Pitman, R. K., Carson, M. A., McMullin, K., Whalen, P., & Makris, N. (2003). Selectively reduced regional cortical volumes in post-traumatic stress disorder. Neuroreport, 14(7), 913-916. https://doi.org/10.1097/01.wnr.0000071767.24455.10; Rogers, M. A., Yamasue, H., Abe, O., Yamada, H., Ohtani, T., Iwanami, A., Aoki, S., Kato, N., & Kasai, K. (2009). Smaller amygdala volume and reduced anterior cingulate gray matter density associated with history of post-traumatic stress disorder. Psychiatry Research: Neuroimaging, 174(3), 210-216. https://doi.org/10.1016/j.pscychresns.2009.06.001; Wrocklage, K. M., Averill, L. A., Scott, J. C., Averill, C. L., Schweinsburg, B., Trejo, M., Roy, A., Weisser, V., Kelly, C., Martini, B., Harpaz-Rotem, I., Southwick, S. M., Krystal, J. H., & Abdallah, C. G. (2017). Cortical thickness reduction in combat exposed US veterans with and without PTSD. European Neuropsychopharmacology, 27(5), 515-525. https://doi.org/10.1016/j.euroneuro.2017.02.010
36 Gilbertson, M. W., Shenton, M. E., Ciszewski, A., Kasai, K., Lasko, N. B., Orr, S. P., & Pitman, R. K. (2002). Smaller hippocampal volume predicts pathologic vulnerability to psychological trauma. Nature Neuroscience, 5(11), 1242-1247. https://doi.org/10.1038/nn958; Villarreal, G., Hamilton, D. A., Petropoulos, H., Driscoll, I., Rowland, L. M., Griego, J. A., Kodituwakku, P. W., Hart, B. L., Escalona, R., & Brooks, W. M. (2002). Reduced hippocampal volume and total white matter volume in posttraumatic stress disorder. Biological Psychiatry, 52(2), 119-125. https://doi.org/10.1016/s0006-3223(02)01359-8; Wang, X., Xie, H., Chen, T., Cotton, A. S., Salminen, L. E., Logue, M. W., Clarke-Rubright, E. K., Wall, J., Dennis, E. L., O’Leary, B. M., Abdallah, C. G., Andrew, E., Baugh, L. A., Bomyea, J., Bruce, S. E., Bryant, R., Choi, K., Daniels, J. K., Davenport, N. D., Davidson, R. J., … Liberzon, I. (2021). Cortical volume abnormalities in posttraumatic stress disorder: An ENIGMA-psychiatric genomics consortium PTSD workgroup mega-analysis. Molecular Psychiatry, 26(8), 4331–4343. https://doi.org/10.1038/s41380-020-00967-1
37 These parts of the brain are the amygdala and prefrontal regions. See: Nicholson, A. A., Densmore, M., Frewen, P. A., Théberge, J., Neufeld, R. W., McKinnon, M. C., & Lanius, R. A. (2015). The dissociative subtype of posttraumatic stress disorder: Unique resting-state functional connectivity of basolateral and centromedial amygdala complexes. Neuropsychopharmacology, 40(10), 2317-2326. https://doi.org/10.1038/npp.2015.79
38 The amygdala-orbitofrontal cortex network showed fewer connections as did the two striatal-subcortical pathways. See: Zhu, X., Helpman, L., Papini, S., Schneier, F., Markowitz, J. C., Van Meter, P. E., Lindquist, M. A., Wager, T. D., & Neria, Y. (2017). Altered resting state functional connectivity of fear and reward circuitry in comorbid PTSD and major depression. Depression & Anxiety, 34(7), 641-650. https://doi.org/10.1002/da.22594
39 Shalev, A. Y., Sahar, T., Freedman, S., Peri, T., Glick, N., Brandes, D., Orr, S. P., & Pitman, R. K. (1998). A prospective study of heart rate response following trauma and the subsequent development of posttraumatic stress disorder. Archives of General Psychiatry, 55(6), 553-559. https://doi.org/10.1001/archpsyc.55.6.553
40 Adenauer, H., Catani, C., Keil, J., Aichinger, H., & Neuner, F. (2010). Is freezing an adaptive reaction to threat? Evidence from heart rate reactivity to emotional pictures in victims of war and torture. Psychophysiology, 47(2), 315-322. https://doi.org/10.1111/j.1469-8986.2009.00940.x
41 Glover, E. M., Phifer, J. E., Crain, D. F., Norrholm, S. D., Davis, M., Bradley, B., Ressler, K. J., & Jovanovic, T. (2011). Tools for translational neuroscience: PTSD is associated with heightened fear responses using acoustic startle but not skin conductance measures. Depression & Anxiety, 28(12), 1058-1066. https://doi.org/10.1002/da.20880
42 Schneider, M., & Schwerdtfeger, A. (2020). Autonomic dysfunction in posttraumatic stress disorder indexed by heart rate variability: A meta-analysis. Psychological Medicine, 50(12), 1937–1948. https://doi.org/10.1017/S003329172000207X
43 Aspesi, D., & Pinna, G. (2018). Could a blood test for PTSD and depression be on the horizon? Expert Review of Proteomics, 15(12), 983-1006. https://doi.org/10.1080/14789450.2018.1544894; Vermetten, E., Baker, D. G., Jetly, R., & McFarlane, A. C. (2016). Concerns over divergent approaches in the diagnostics of posttraumatic stress disorder. Psychiatric Annals, 46(9), 498-509. .https://doi.org/10.3928/00485713-20160728-02
44 Alzubi, J., Nayyar, A., & Kumar, A. (2018). Machine learning from theory to algorithms: An overview. Journal of Physics: Conference Series, https://doi.org/10.1088/1742-6596/1142/1/012012
45 Ibid.
46 Dean, K. R., Hammamieh, R., Mellon, S. H., Abu-Amara, D., Flory, J. D., Guffanti, G., Wang, K., Daigle Jr, B. J., Gautam, A., & Lee, I. (2020). Multi-omic biomarker identification and validation for diagnosing warzone-related post-traumatic stress disorder. Molecular Psychiatry, 25(12), 3337-3349. https://doi.org/10.1038/s41380-019-0496-z
47 Abi‐Dargham, A., Moeller, S. J., Ali, F., DeLorenzo, C., Domschke, K., Horga, G., Jutla, A., Kotov, R., Paulus, M. P., & Rubio, J. M. (2023). Candidate biomarkers in psychiatric disorders: State of the field. World Psychiatry, 22(2), 236-262. https://doi.org/10.1002/wps.21078
48 Galatzer-Levy, I. R., & Bryant, R. A. (2013). 636,120 ways to have posttraumatic stress disorder. Perspectives on Psychological Science, 8(6), 651-662. https://doi.org/10.1177/1745691613504115
49 Ryder, A. L., Azcarate, P. M., & Cohen, B. E. (2018). PTSD and physical health. Current Psychiatry Reports, 20, 1-8.; Walter, K. H., Levine, J. A., Highfill‐McRoy, R. M., Navarro, M., & Thomsen, C. J. (2018). Prevalence of posttraumatic stress disorder and psychological comorbidities among U.S. active duty service members, 2006–2013. Journal of Traumatic Stress, 31(6), 837-844. https://doi.org/10.1007/s11920-018-0977-9
50 Abi‐Dargham, A., Moeller, S. J., Ali, F., DeLorenzo, C., Domschke, K., Horga, G., Jutla, A., Kotov, R., Paulus, M. P., & Rubio, J. M. (2023). Candidate biomarkers in psychiatric disorders: State of the field. World Psychiatry, 22(2), 236-262. https://doi.org/10.1002/wps.21078
51 Ibid.
52 Abbasi, J. (2019). First biomarker-based screening tool for PTSD. JAMA, 322(15), 1437-1437. https://doi.org/10.1001/jama.2019.15339
53 Rush, A. J., & Ibrahim, H. M. (2018). Speculations on the Future of Psychiatric Diagnosis. The Journal of Nervous and Mental Disease, 206(6), 481–487. https://doi.org/10.1097/NMD.0000000000000821
54 Lehrner, A., & Yehuda, R. (2014). Biomarkers of PTSD: military applications and considerations. European Journal of Psychotraumatology, 5. https://doi.org/10.3402/ejpt.v5.23797
55 Galatzer-Levy, I. R., Steenkamp, M. M., Brown, A. D., Qian, M., Inslicht, S., Henn-Haase, C., Otte, C., Yehuda, R., Neylan, T. C., & Marmar, C. R. (2014). Cortisol response to an experimental stress paradigm prospectively predicts long-term distress and resilience trajectories in response to active police service. Journal of Psychiatric Research, 56, 36-42. https://doi.org/10.1016/j.jpsychires.2014.04.020; Michopoulos, V., Norrholm, S. D., & Jovanovic, T. (2015). Diagnostic biomarkers for posttraumatic stress disorder: Promising horizons from translational neuroscience research. Biological Psychiatry, 78(5), 344-353. https://doi.org/10.1016/j.biopsych.2015.01.005
56 Yehuda, R., Pratchett, L. C., Elmes, M. W., Lehrner, A., Daskalakis, N. P., Koch, E., Makotkine, I., Flory, J. D., & Bierer, L. M. (2014). Glucocorticoid-related predictors and correlates of post-traumatic stress disorder treatment response in combat veterans. Interface Focus, 4(5), 20140048. https://doi.org/10.1098/rsfs.2014.0048
57 Nijdam, M. J., Van Amsterdam, J. G. C., Gersons, B. P. R., & Olff, M. (2015). Dexamethasone-suppressed cortisol awakening response predicts treatment outcome in posttraumatic stress disorder. Journal of Affective Disorders, 184, 205-208. https://doi.org/10.1016/j.jad.2015.05.058
58 Rauch, S. A., King, A. P., Abelson, J., Tuerk, P. W., Smith, E., Rothbaum, B. O., Clifton, E., Defever, A., & Liberzon, I. (2015). Biological and symptom changes in posttraumatic stress disorder treatment: A randomized clinical trial. Depression & Anxiety, 32(3), 204-212. https://doi.org/10.1002/da.22331
59 Ramírez, C. G., Queijeiro, A. V., Morales, S. J., López, D. B., Miranda, A. H., Chow, A. R., Cárdenas, L. T., & Estrada, M. G. (2020). The NR3C1 gene expression is a potential surrogate biomarker for risk and diagnosis of posttraumatic stress disorder. Psychiatry Research, 284, 112797. https://doi.org/10.1016/j.psychres.2020.112797
60 Wang, Q., Shelton, R. C., & Dwivedi, Y. (2018). Interaction between early-life stress and FKBP5 gene variants in major depressive disorder and post-traumatic stress disorder: A systematic review and meta-analysis. Journal of Affective Disorders, 225, 422-428. https://doi.org/10.1016/j.jad.2017.08.066
61 Li, L., Bao, Y., He, S., Wang, G., Guan, Y., Ma, D., Wang, P., Huang, X., Tao, S., & Zhang, D. (2016). The association between genetic variants in the dopaminergic system and posttraumatic stress disorder: A meta-analysis. Medicine, 95(11). https://doi.org/10.1097/MD.0000000000003074
62 Ibid.
63 Lind, M. J., Marraccini, M. E., Sheerin, C. M., Bountress, K., Bacanu, S. A., Amstadter, A. B., & Nugent, N. R. (2017). Association with rs2267735 in the ADCYAP1R1 gene: A meta-analysis. Journal of Traumatic Stress, 30(4), 389-398. https://doi.org/10.1002/jts.22211; Ressler, K. J., Mercer, K. B., Bradley, B., Jovanovic, T., Mahan, A., Kerley, K., Norrholm, S. D., Kilaru, V., Smith, A. K., & Myers, A. J. (2011). Post-traumatic stress disorder is associated with PACAP and the PAC1 receptor. Nature, 470(7335), 492-497. https://doi.org/10.1038/nature09856
64 Uddin, M., Ratanatharathorn, A., Amstrong, D., Kuan, P. F., Aiello, A. E., Bromet, E. J., & Smith, A. (2018). Epigenetic meta-analysis across three civilian cohorts identifies NGR1 and HGS as blood-based biomarkers for post-traumatic stress disorder. Epigenomics, 10(12), 1585-1601. https://doi.org/10.2217/epi-2018-0049
65 Ganzel, B. L., Kim, P., Glover, G. H., & Temple, E. (2008). Resilience after 9/11: Multimodal neuroimaging evidence for stress-related change in the healthy adult brain. Neuroimage, 40(2), 788-795. https://doi.org/10.1016/j.neuroimage.2007.12.010; Rogers, M. A., Yamasue, H., Abe, O., Yamada, H., Ohtani, T., Iwanami, A., Aoki, S., Kato, N., & Kasai, K. (2009). Smaller amygdala volume and reduced anterior cingulate gray matter density associated with history of post-traumatic stress disorder. Psychiatry Research: Neuroimaging, 174(3), 210-216. https://doi.org/10.1016/j.pscychresns.2009.06.001
66 Xie, H., Claycomb Erwin, M., Elhai, J. D., Wall, J. T., Tamburrion, M. B., Brickman, K. R., Kaminski, B., McLean, S. A., Liberzon, I., & Wang, X. (2018). Relationship of hippocampal volumes and posttraumatic stress disorder symptoms over early posttrauma periods. Biological Psychiatry Cognitive Neuroscience & Neuroimaging, 3(11), 968-975. https://doi.org/10.1016/j.bpsc.2017.11.010
67 Wang, X., Xie, H., Chen, T., Cotton, A. S., Salminen, L. E., Logue, M. W., Clarke-Rubright, E. K., Wall, J., Dennis, E. L., O’Leary, B. M., Abdallah, C. G., Andrew, E., Baugh, L. A., Bomyea, J., Bruce, S. E., Bryant, R., Choi, K., Daniels, J. K., Davenport, N. D., Davidson, R. J., … Liberzon, I. (2021). Cortical volume abnormalities in posttraumatic stress disorder: An ENIGMA-psychiatric genomics consortium PTSD workgroup mega-analysis. Molecular Psychiatry, 26(8), 4331–4343. https://doi.org/10.1038/s41380-020-00967-1
68 Nicholson, A. A., Densmore, M., Frewen, P. A., Théberge, J., Neufeld, R. W., McKinnon, M. C., & Lanius, R. A. (2015). The dissociative subtype of posttraumatic stress disorder: Unique resting-state functional connectivity of basolateral and centromedial amygdala complexes. Neuropsychopharmacology, 40(10), 2317-2326. https://doi.org/10.1038/npp.2015.79
69 Zhu, X., Helpman, L., Papini, S., Schneier, F., Markowitz, J. C., Van Meter, P. E., Lindquist, M. A., Wager, T. D., & Neria, Y. (2017). Altered resting state functional connectivity of fear and reward circuitry in comorbid PTSD and major depression. Depression & Anxiety, 34(7), 641-650. https://doi.org/10.1002/da.22594
70 Shalev, A. Y., Sahar, T., Freedman, S., Peri, T., Glick, N., Brandes, D., Orr, S. P., & Pitman, R. K. (1998). A prospective study of heart rate response following trauma and the subsequent development of posttraumatic stress disorder. Archives of General Psychiatry, 55(6), 553-559. https://doi.org/10.1001/archpsyc.55.6.553
71 Glover, E. M., Phifer, J. E., Crain, D. F., Norrholm, S. D., Davis, M., Bradley, B., Ressler, K. J., & Jovanovic, T. (2011). Tools for translational neuroscience: PTSD is associated with heightened fear responses using acoustic startle but not skin conductance measures. Depression & Anxiety, 28(12), 1058-1066. https://doi.org/10.1002/da.20880
About the Author
Soonjo Hwang is Associate Professor, Research Director, and Anna O. Stake Professor at the University of Nebraska Medical Center Department of Psychiatry. He specializes in neuroscience, neuroimaging, and phenotyping of various psychopathologies, including emotional and behavioral dysregulation, developmental trajectory of brain function, and clinical trials of novel treatment options.
Suggested Citation
Hwang, S. (2024). Exploring biomarker technology to enhance the diagnosis and treatment of PTSD in justice-involved veterans. Council on Criminal Justice. https://counciloncj.org/exploring-biomarker-technology-to-enhance-the-diagnosis-and-treatment-of-ptsd-in-justice-involved-veterans/